As featured in Waterline Summer 2023
Chelants in water treatment:
An overview
By Kathy Hood BSc (hons), CChem, MRSC – WCS Group
Water treatment chemicals for many applications including chemical cleaning, scale inhibition and wastewater treatment contain chelants. The effective use of chelants is particularly critical when considering the topics of scale inhibition and the effective use of iron plateau levels when undertaking pre-commission cleaning or flushing closed circuit systems. The aim of this article is to provide some background to the science behind chelants and to give a greater understanding of their selection and use in water treatment.
Chelants, also known as chelating agents, are organic compounds that can form stable, water-soluble complexes with metal ions. These complexes are held together in a way (by coordinate covalent bonds), that prevents the metal ions from reacting with other substances in the water.
The term chelant was first used in 1920 by Sir Gilbert T. Morgan and Harry D. K. Drew. The word comes from the Greek word for claw and illustrates how a chelant forms a complex where two or more complexing groups (ligands) bond to a metal incorporating it into a ring type structure:

Effectively, a chelant (for example EDTA/NTA) “traps” the metal ion, inhibiting it from reacting with other ions. The resulting complex is known as a chelate. Although, often used interchangeably with the term chelant, sequestrants can bind to several metal ions simultaneously, whereas a chelant molecule binds to just one.
This article gives an overview of the main types of chelants (and sequestrants), focusing on those characteristics which have practical relevance to water treatment. [1, 2, 3, 4]. It will also touch on sustainability and the drive to “greener” chelants.
Chelants
We may think that chelants are chemicals used within formulations to chelate with metals, but in fact they are all around us and often found in nature. For example, the green colour in plants is from chlorophyll, a chelate of magnesium.
Within water treatment, there are several types of chelants, some, of which are described below. Throughout this article, I have referred to the chelants as the acid but in practice, salts are typically used dependant on the pH and solubility.
Generally, when we think of chelants in water treatment, the first ones we think of are EDTA and NTA which belong to a class of chemicals generically described as aminopolycarboxylic acids:

These molecules contain both amine and carboxylic acid groups, both of which can bind to metal ions. Some metal ions complex more strongly to nitrogen (from the amine) and others to oxygen (from the carboxylic acid). The number of potential binding sites allows for the metal to be bound strongly producing a stable complex:

Chelants coordinate with metal ions (e.g. Fe3+, ferric or Fe2+, ferrous iron) and not with metals in their free metallic (un-ionized) state (e.g. iron).
The stability of the chelate complex formed is the reason why this type of chelant (aminopolycarboxylic acids), including EDTA (ethylenediaminetetraacetic acid), NTA (nitrilotriacetic acid), and DTPA (diethylenetriaminepentaacetic acid), are widely used in various water treatment applications.
The specific choice of aminopolycarboxylic acid (NTA, EDTA, DTPA etc.) to be used will depend on various factors including the pH of the system, how oxidising the environment is and the type of metal ion to be chelated. There are published stability constants for various metal/chelating agent combinations. The higher the value, the more stable the complex formed.
Some examples of stability constants at 20oC are given in the table on the next page [5].
The order in which metals will be chelated can be predicted by looking at the stability constants with those metal ions producing the most stable complexes being chelated preferentially. This means that if you look at the above table, in a system containing Fe3+, Cu2+, Fe2+ and Ca2+ any EDTA added will complex the Fe3+ first, before complexing with the Cu2+ and then the Fe2+. The Ca2+ will be the last to be complexed (assuming sufficient EDTA is available).
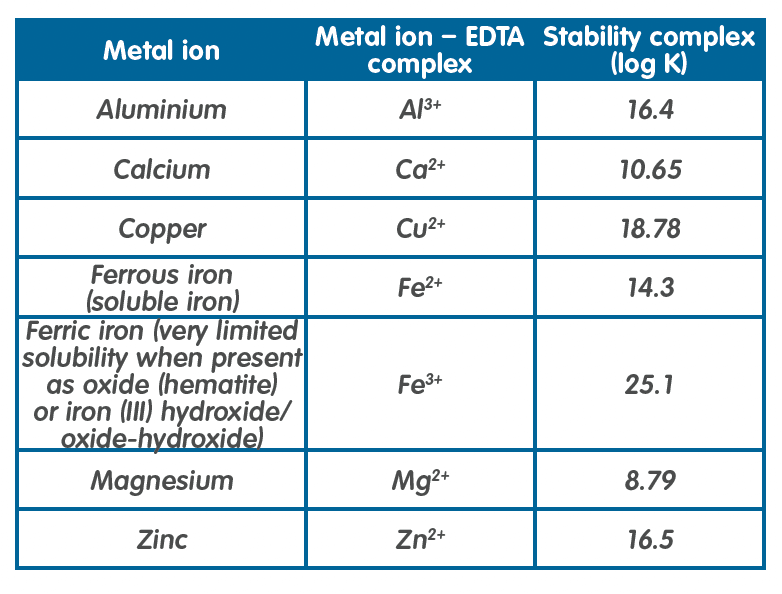
The stability constant can also be used to indicate whether a metal is likely to be displaced from its chelate complex by another metal. For instance, if you have a solution of a calcium-EDTA complex, and add a copper ion to that solution, the copper will displace the calcium giving you copper-EDTA chelate. The pH will be a factor in this process.
Stability constants are a measure of how tightly the metal is bound to the chelating agent indicating how stable the complex will be. Other factors such as pH, temperature, ionic strength, oxidising conditions, and the presence of other competing ligands will affect the stability and efficacy of any chelant/metal ion system; sulphide in particular, can have a significant effect. Therefore, the values in the above table should be taken as a general indication rather than as a precise guide under all conditions. Conditional stability constants may be used to look at the effect of other parameters, particularly pH.
Whilst stability constants will typically be lower at higher temperatures, the effect is not generally significant. The reaction between free divalent metal ions (2+) and aminopolycarboxylic acids in solution is very rapid although it is slower with trivalent ions (3+). Solubility also affects the rate of reaction with the rate being much slower with solid metallic salts. If you have a mixture of calcium sulphate and ferric oxide (corrosion deposits – Fe3+), even though the iron forms a more stable chelate than calcium, the calcium will be chelated first because the calcium sulphate is more soluble than ferric oxide.
Both metal ions and the chelants are affected by pH. As the pH is decreased and the concentration of protons (hydrogen ions) increases, there comes a point where protons will outcompete the metal ions to bind with the chelant. Similarly, as the pH is increased and the concentration of hydroxide ions increases, hydroxide ions will eventually out compete the metal ions to bind with the chelant. The pH at which the protons/hydroxide ions out compete the metal ions will differ depending on the metal. If we look at EDTA, it will optimally chelate calcium and the other alkaline earth metals at a pH greater than 6, ferric iron at pH less than 8 and most other heavy metals at a pH less than 12.
For calcium, protons, (H+ ions), will outcompete the calcium ions for complexing with the EDTA at a pH less than 6. However, for most common divalent (2+) heavy metals, the competition from protons is not an issue at lower pHs. Generally, the effect of pH is more marked at higher values (neutral to alkaline) as many common metal hydroxides have limited solubility. If you raise the pH of a ferric-EDTA (Fe3+) solution, you will start to get precipitation of ferric hydroxide at pH 8. By the time you get to pH 12, the ferric-EDTA complex will be completely converted to free EDTA and ferric hydroxide.
Suppliers of aminopolycarboxylic acids (chelants) provide information on the calculated (theoretical) weight of chelant needed to chelate a specific amount of metal. These theoretical calculations are based on one molecule of chelant reacting with one metal ion. The calculation is done based on the relevant molecular weights.
For example, the molecular weight of EDTA is 292 and that of iron is 56. From this calculation the amount of EDTA required to complex 1g iron is approximately 5g. (If 1g of EDTA.4Na is used, the amount required will be approximately 8g/g iron).
Phosphonates
Phosphonates are also commonly used to bind with metal ions. These molecules, including HEDP (hydroxyethylidene diphosphonic acid), PBTC (phosphonobutane-1,2,4- tricarboxylic acid) and ATMP (aminotri(methylene phosphonic acid)), contain both phosphonic acid and carboxylic acid groups, which have a high affinity for metal ions:
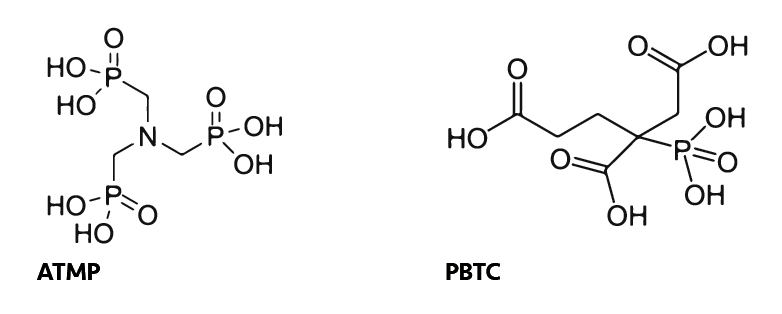
The stability of ATMP-metal complexes generally follows the order Fe3+ > Al3+ > Ca2+ > Mg2+ with Fe3+ complexes being the most stable due to the high affinity of Fe3+ for the phosphonate ligands.
There are many different phosphonates available with variations in stability constants reported. In general, at acid or neutral pH, phosphonates have lower stability constants with heavy metal ions than aminopolycarboxylic acid chelants. This is not necessarily the case at higher pH although this does depend on the specific phosphonate.
Many phosphonates are sequestrants rather than chelants and are able to sequester several moles of a metal ion for each mole of phosphonate. They are used in many formulations to prevent scale deposition, particularly in the control of calcium salt deposition. The concentration of calcium salts in the system is not generally the critical factor if it is kept below the level at which precipitation of calcium salts (scaling) will occur. For this application, it is generally more cost effective to use phosphonates rather than an aminopolycarboxylic acid chelant. On a weight for weight basis, a phosphonate can complex with a greater amount of a metal ion than the comparative weight of a chelant such as EDTA.
Other factors are important when deciding on the best phosphonate to use including the calcium tolerance of the phosphonate. PBTC has better calcium tolerance than ATMP which is still better than HEDP. Stability of the phosphonate molecule in the presence of oxidising biocides such as chlorine and bromine is particularly important when formulating cooling tower scale and corrosion inhibitors. PBTC has better chlorine stability than HEDP or ATMP.
In addition to their sequestrant capability, phosphonates can also act as crystal growth inhibitors, preventing existing scale crystals from growing larger and forming a solid layer, as well as disrupting the formation of new crystals. This mechanism allows a low concentration of a phosphonate to manage a significantly larger concentration of calcium hardness, which is why they are known as threshold inhibitors.
Polymer-based sequestrants / chelants
These are relatively low molecular weight water soluble polymers that can bind metal ions through multiple coordination sites. Examples include polyacrylic acid and PA (Phosphonic acid)/AMPS (2-Acrylamido-2-methylpropane sulfonic acid). Typically, the molecular weight of such polymers is of the order of 1000-10000g/mol.
Although these polymers have many potential binding sites for metal ions, they typically form less stable complexes than aminopolycarboxylic acid chelants such as EDTA. At ambient temperature the stability constant for a calcium – polyacrylate complex is considerably lower than for the calcium-EDTA complex. This is thought to be because the binding sites are fairly distant, and the resulting metal-polymer complexes may comprise large rings. At higher temperatures, polyacrylates can form stronger complexes with metal ions than other chelants. This is particularly important in specific high temperature applications such as steam boilers.
Polyacrylates are effective chelating agents because they contain many carboxylic acid groups (-COOH) along their polymer chains, which can bond with metal ions. This also makes polyacrylates highly water-soluble.
PA/AMPS are effective chelants for metal ions such as calcium, magnesium, iron, and aluminium. In very high concentrations of calcium, polyacrylates are liable to form an uncharged calcium polyacrylate complex that will precipitate out of solution. However, the addition of the sulphonate group in PA/AMPS prevents this.
PA and AMPS are often used in combination with other chelating agents / sequestrants to provide a more complete solution for complex industrial water treatment problems.
Choosing a chelating agent
There are many factors to be considered when choosing a chelating agent (or sequestrant) including: cost effectiveness, the metal you want to chelate, the stability of the chelant and resulting metal chelate, and the environment in which the chelant will be used.
Chelants are organic compounds which will, at some point, undergo thermal degradation. When chelants degrade, they can release bound metal ions, which can react with other compounds or surfaces and potentially cause corrosion.
The corrosiveness of degraded chelants will vary depending on the type of chelant, the concentration of the degraded chelant, and the materials it comes into contact with. Under certain (fairly extreme) conditions, some chelants, such as EDTA, can be corrosive to metals like copper and zinc, while others, such as NTA, are less corrosive. The presence of oxygen increases the potential for corrosion as either the aminopolycarboxylic acid or the resulting chelate may degrade.
Polyacrylates are less corrosive than EDTA/NTA. Phosphonates such as ATMP, HEDP, and PBTC have good hydrolytic and thermal stability but under certain conditions, phosphonates can undergo hydrolysis to form phosphates which may precipitate out of solution. The stability of phosphonate-metal complexes will depend on the metal and phosphonates used, as well as system conditions. In general, HEDP-metal complexes are less stable than ATMP-metal complexes, which are less stable than PBTC-metal complexes.
Chelants and sustainability
When it comes to sustainability, the use of chelants can have both positive and negative effects. They can help to reduce the environmental impact of certain industries by allowing more efficient use of water and energy. For example, chelants can be used to remove heavy metals from wastewater thus reducing the amount of pollutants released into the environment.
Dithiocarbamates are used in wastewater treatment as chelants and can be used either in addition to the traditional techniques to scavenge any metals remaining after precipitation steps or can be used instead of the traditional technologies entirely without the production of large quantities of sludge.
However, the production and use of some chelants can have negative environmental impacts as some chelants are derived from non-renewable resources and require significant energy inputs to produce. Additionally, some chelants / sequestrants can persist in the environment. Aminopolycarboxylic acids can destroy bacterial cell membranes and harm plants by increasing the uptake of toxic heavy metals. There is also the potential for metals, some of which may be toxic, to be remobilised from sediments and aquifers which may pose a risk to drinking and ground water.
Although NTA is biodegradable, it is a suspected carcinogen. EDTA like some other commonly used chelants are not readily degradable and will persist in the environment. [6, 7].
The presence of nitrogen and/or phosphorus can lead to eutrophication and consequently algal blooms. However, it is claimed that because phosphonates degrade slowly, they will not significantly contribute to the rapid growth of algae.
There is growing interest in developing chelants that are more environmentally friendly and biodegradable, making them a more sustainable alternative to traditional chelants.
Tannins are increasingly used in wastewater treatment as an effective but more sustainable alternative to traditional coagulants based on aluminium and iron salts. Tannins are biodegradable polymers derived from natural plant-based sources. They contain a large number of hydroxide groups which enables them to chelate metals very effectively. As coagulants they have been very successfully used to reduce turbidity, colour, suspended solids, and organic matter from wastewater streams. Furthermore, the use of tannins can result in cost savings, when compared to an aluminium based product, it has been found that for the same level of treatment, around half the quantity of sludge will be produced.
Citric acid has been used as a chelant for some time but has stability constants significantly lower than does EDTA and lower than other biodegradable chelants such as salicyclic acid for iron. [8]
There are other “bio-inspired chelants” which as the term suggests are influenced by biological molecules. Examples of such biodegradable chelants include glutamic acid diacetate, a derivative of an amino acid. Experimental data suggests that a comparison of the chelating power of EDTA products and glutamic acid diacetate products are similar for many metal ions including Ca(II) and Fe(III). [2]
Chelants based on the structure of proteins include polyaspartic acid and polyglutamic acid. Polyaspartic acid is claimed to be a biodegradable alternative to polyacrylates with good calcium tolerance which is achieved by its ability to disperse calcium carbonate rather than its action as a chelant. Iminodisuccinic acid is another biodegradable chelant and is reported to have good chelating properties particularly for calcium and stability over a wide pH range. It is more applicable to use in detergents than in water treatment although a substituted version, hydroxy iminodisuccinic acid is also available. However, slightly higher stability constants have been reported for metal-glutamic acid diacetate complexes than for the corresponding hydroxy iminodisuccinic acid complexes, although these are still lower than for EDTA. [9]
Glucoheptonate is a glucose derivative which is a sequestrant rather than a chelant. Although it generally has lower stability constants than EDTA, it performs better at higher pHs (9-11) with many metal ions including Fe(III). [1]
Research continues with an ongoing focus on sustainability. This includes the development of anti-scale products where the active component(s) are derived from plants, as well as products for cleaning applications and wastewater treatment.
These new chelant formulations hold promise for improving the efficiency and sustainability of water treatment processes, and reducing the environmental impact of industrial activities that rely on chelants. The performance of many of these chelants is proven and market/economic factors will be the driver for their future growth.
In summary, there are many different chelating / sequestering agents with various properties. Unfortunately, there is no perfect chelant suitable for all applications. The right choice of chelant / sequestrant for any particular application therefore needs careful thought. Water treatment requires a holistic viewpoint and very often chelants / sequestrants will need to be incorporated into a formulation to provide the balance of properties required for a particular water system. This is where the expertise of your water treatment chemical provider is invaluable in ensuring you get the right product for your needs.
References
[1] Nouryon, “Chelates Product Guide,” 2021. [Online].
Available: https://www.nouryon.com/globalassets/inriver/resources/brochure-homecare-dissolvine-product-guideglobal-en.pdf.
[2] Nouryon, “Dissolvine® GL technical brochure,” 2022. [Online]. Available: https://www.nouryon.com/globalassets/inriver/resources/brochure-chelates-dissolvinegl-global-en.pdf.
[3] Dow, “General concepts of the chemistry of chelation,” 2021. [Online]. Available: https://www.dow.com/content/dam/dcc/documents/en-us/app-tech-guide/113/113-01388-01-chelation-chemistry-general-concepts-of-the-chemistryof-chelation.pdf.
[4] Emily R. Clark, Lubrizol, “A Brief Review of Deposit Control Polymers in Industrial Water,” [Online]. Available: https://www.lubrizol.com/-/media/Lubrizol/Water-Treatment/Documents/Carbosperse-Library/A-Brief-Reviewof-DCPs-in-Industrial-Water-Treatment_AWT2018_ClarkER.pdf.
[5] R. S. A.E. Martell, “NIST Critically selected stability constants of metal complexes,” NIST standard reference database 46, Version 7.0,, 2003.
[6] T. Almubarak, J. Ng, R. Ramanathan and H. A. Nasr-El-Din, “From initial treatment design to final disposal of chelating agents: a review of corrosion and degradation mechanisms.,” RSC Adv., vol. 12, no. 3, pp. 1813-1833, 2022.
[7] B. Nowack and J. M. VanBriesen, “Chelating Agents in the Environment,” 2005. [Online]. Available: https://pubs.acs.org/doi/pdf/10.1021/bk-2005-0910.ch001.
[8] G. Mudlagiri and P. Manju, “Effects of chelating agents on protein, oil, fatty acids, and minerals in soybean see.,” Agricultural Sciences, vol. 3, no. 4, pp. 517-523, 2012.
[9] Z. A. Begum., I. M. M. Rahman, H. S. Sawai, Y. Tate, T. M. Maki and H. Hasegawa, “Stability Constants of Fe(III) and Cr(III) Complexes with dl-2-(2-Carboxymethyl)nitrilotriacetic Acid (GLDA) and 3-Hydroxy-2,2′-iminodisuccinic acid (HIDS) in Aqueous Solution,” J. Chem. Eng. Data, vol. 57, no. 10, p. 2723–2732, 2012.