WATERLINE ONLINE EXCLUSIVE ARTICLE

The Need to Understand the Risks and Prevent Aspiration of Pathogens in Healthcare Premises
Elaine Waggott (AMRSPH)
The need to reduce the risks of splashing and airborne pathogenic aerosol has never been more important. In the wake of Covid-19 recent research findings have starting to confirm what many already suspected – that Covid-19 acts like many existing pathogens in the ways it can be transmitted via water-borne particles and poor hand hygiene.
Unacceptable levels of sickness and deaths have long been associated with poor hand hygiene, close contact with infected people and inadequate cleaning. In many countries, initiatives addressing education, cleaning and audit, together with compulsory reporting of infections, have brought about benefits leading (in some cases) to the reduction of headline rates of infections, such as methicillin-resistant Staphylococcus aureus (MRSA), Clostridium difficile (C.dif) and Legionnaires’ Disease. It is readily accepted now, that a common mode of transmission is contact between the patient, the staff and the environment.
Inappropriate hand hygiene practice has been identified as a significant contributor to numerous outbreaks. Several studies have shown the impact of improved hand hygiene on the risk of healthcare associated infection and multi-resistant pathogen cross-transmission. To date, most studies have focused on methicillin resistant Staphylococcus aureus.
Bacteria present on human skin can be considered as belonging to one of two groups: resident and transient flora. Transient flora colonises the superficial layers of the skin. It has a short-term persistence on skin, but a high pathogenic potential. It is usually acquired by healthcare workers during direct contact with patients or contaminated environmental surfaces adjacent to the patient, and is responsible for most healthcare associated infections and spread of antimicrobial resistance resulting from cross-transmission. Resident flora is attached to deeper skin layers and has a low pathogenic potential unless introduced into the body by invasive devices. It is also more difficult to remove mechanically. Hand hygiene decreases colonisation with transient flora and can be achieved either through hand washing or hand antisepsis.[1]
Whilst this and other studies have shown that outbreaks can be reduced by improved hand hygiene compliance and better cleaning of the environment. Transmission of infection by the air has often been less well investigated, leading sometimes to a complacency in this mode of transmission.
It has been proven already that Norovirus can be transmitted by aerosol and is difficult to contain in a hospital ward without sufficient single rooms with en-suite toilets. Now with the additional battle against Covid-19, the transmission by aerosol now needs to be urgently considered and the risks substantially reduced.
Although the direct transmission from infected person/s is the primary source of aerosols and droplets, other scenarios such as medical procedures, surgeries [2], fast-running tap water and toilet flushes [3] also generate aerosols contaminated with infectious pathogens. The most common types of viruses causing infections in the respiratory tract through aerosol transmission are influenza viruses, rhinoviruses, coronaviruses, respiratory syncytial viruses (RSVs), and parainfluenza viruses [3,4]. Some articles have postulated three modes in which the influenza virus can be transmitted: aerosol transmission, droplet transmission, and self-inoculation of the nasal mucosa by contaminated hands.[2]
Historically, natural ventilation was seen to be beneficial in hospital wards and was part of hospital design. With the advent of sealed high-rise buildings and forced ventilation, expensive negative pressure rooms have been sometimes been introduced to house patients with infections thought likely to be transmitted by aerosol.
Aerosols can be defined as liquid or solid particles suspended in the air by humans, animals, instruments, or machines. Bio-aerosols are aerosols consisting of particles of any kind of organism [1, 2]. The characteristics of bio-aerosols differ depending on environmental influences such as humidity, air flow, and temperature. Aerosols, which are responsible for the transmission of airborne micro-organisms by air, consist of small particles named droplet nuclei (1–5μm) or droplets (>5μm). Droplet nuclei can stay airborne for hours, transport over long distances and contaminate surfaces by falling down. In a review article from 2006 (Tang, Li, Eames, Chan, & Ridgway, 2006), the authors found for SARS‐CoV‐1 that “particles of diameters 1–3 µm remained suspended almost indefinitely, 10 µm took 17 min, 20 µm took 4 min, and 100 µm took 10 seconds to fall to the floor” (Tang et al., 2006). This article notes that aerosol transmission is a well‐known and important exposure pathway for infectious agents such as influenza and other viruses including coronaviruses. As discussed in this article, SARS‐CoV‐1 viral RNA was found in air samples, and long‐range aerosol transport was implicated as the cause of the spread of the disease in several studies.[5]
It has been proven that droplets can contaminate surfaces in a range of over 2 meters [7]. The droplets are also capable of penetrating deep into the lungs, offering a potential route of infection [8]. The susceptibility of acquiring an infectious agent is determined by factors such as: virulence; dose; and pathogenicity of the micro-organism; and the host’s immune response [8-10]. Humans generate bio-aerosols by talking, breathing, sneezing or coughing [6]. Based on the infectious status of a person, the bio-aerosols can contain pathogens including influenza [11, 12], Mycobacterium tuberculosis [8], Staphylococcus aureus, Varicella Zoster Virus, Streptococcus spp. or Aspergillus spp. [12]. Moreover, bio-aerosols can be generated by devices such as ventilation systems, showers, taps and toilets. Showers and tap water are also able to spread environmental microbes such as Legionella spp. [9, 10, 14].
It is now commonly accepted that bio-aerosols containing harmful pathogens are very much a common and serious contributor to healthcare-acquired infections but are these connections new and what was known of such threats in the past?
In 1981 Cordes et al found that Legionella pneumophila serogroup 6 was present in 9 of 16 shower heads in a Chicago hospital ward where 3 patients had contracted Legionnaires’ disease caused by serogroup 6 L. pneumophila. Each patient had showered there 2 to 10 days before the onset of disease symptoms. They also isolated the bacteria in 2 other hospitals and found the same serogroups as had been causing Legionnaires’ disease in those hospitals: serogroup 1 in Pittsburgh and serogroups 1 and 4 in Los Angeles. However, showers from hospital wards where no patients had contracted Legionnaires’ disease also yielded L. pneumophila. The question of whether aerosols of shower water or other exposures to potable water containing L. pneumophila may cause nosocomial Legionnaires’ disease was not proven but they suggested that is definitely deserved further study. [16]
In 1985 Meenhorst et al conducted an investigation into an outbreak of nosocomial legionella pneumonia. It revealed that the potable water of the hospital was contaminated with a distinctive strain of Legionella pneumophila (designated the Le-l strain), which was by culture and serology linked with human illness. To examine the possible role of this water in the outbreak, eight healthy guinea pigs were exposed to an aerosol of concentrated potable water. Seven animals developed pneumonia due to the Le-l strain of L. pneumophila. In one of these guinea pigs, the Le-l strain was also isolated from the blood and spleen. These results provided support to the theory that exposure of humans to aerosols of potable water contaminated with L. pneumophila may cause legionella pneumonia. [17]
Again in 1985 Garye Bollin et all conducted an air sampling test in showers and sink areas at the Youngstown Hospital. A total of two paired water and air samples were obtained from each of the two shower rooms. All four water cultures grew L.pneumophila. Low numbers of aerosolized L. pneumophila (3 to 5 CFU/15 ft3 [0.43m3] of air) were recovered when the air was sampled above the shower doors with the six-stage sampler. Equal numbers of organisms were recovered in the first and second 15-min sampling periods.
A total of 19 paired water and air samples were obtained from 14 hot-water taps. A total of 17 of the water cultures grew L. pneumophila. Two colonies of L. pneumophila (one on stage 1, one on stage 3) were recovered from air around one of the three taps tested with the six-stage unit. A total of 11 colonies were recovered (6 on stage1, 5 on stage 2) from 5 of the remaining 13 taps tested with the two-stage unit. All positive air cultures from the two-stage unit were obtained during the period when the tap water was running. None were ever obtained before the tap water was turned on or after it was turned off. No air cultures were positive more than once among the rooms that were tested two and three times. [18]
More recently at the University Hospital of Brussels the incidence of new patients with Carbapenemase-producing Enterobacteriaceae (CPE) rose from 1 case in 2010 to 35 cases in 2015. Environmental samples suggested that a contaminated washbasin was the source of the outbreak. Besides other strains, Citrobacter freundii type OXA-48 was frequently isolated from patients and washbasins. To investigate the relationship between those strains, pulsed-field gel electrophoresis was performed. The strains isolated from patients and the washbasin in the implicated room were highly related and pointed to sink-to-patient transmission. In total, 7 of 8 sinks in the isolation rooms of the ICU were found to be CPE contaminated. To control the outbreak, the washbasins and their traps and pipework were replaced with new ones, they were then flushed every morning with a glucoprotamin solution and routines regarding sink practices were improved leading to discontinuation of the outbreak. [19]
Showers, taps and washbasins are not the only means of transmission via aerosol. Toilet Flushing is another large risk area.
Bio-aerosol production during toilet flushing was first reported in the 1950s by Jessen [15] who “seeded” several types of toilets with Serratia marcescens (then termed Bacillus prodigiosus) and measured bio-aerosols produced by flushing. Agar-filled “settle plates” caught bio-aerosols that fell out of the air because of gravity, and a Bourdillon slit impactor collected air samples. Cistern-fed, gravity-flow toilets and a mains-fed pressure-valve toilet were examined. In addition to colonies found on the floor-based settle plates, microbes were still being captured from the air 8 minutes after the flush, indicating collection of “droplet nuclei” bio-aerosols. Droplet nuclei are the tiny particles that remain after the water in a droplet evaporates. They have negligible settling velocity and will float with natural air currents. Jessen observed that the amount of bio-aerosol increased with increasing flush energy.
In 2005 Barker et al experimented with toilet bowls and found that although a single flush reduced the level of micro-organisms in the toilet bowl water when contaminated at concentrations reflecting pathogen shedding, large numbers of micro-organisms persisted on the toilet bowl surface and in the bowl water which were disseminated into the air by further flushes. [21]
In 2011 Best et al [22] performed in-situ testing, using faecal suspensions of C. difficile to simulate the bacterial burden found during disease, to measure C. diff aerosolisation. They also measured the extent of splashing occurring during flushing of two different toilet types commonly used in hospitals.
Their findings were as follows: C. diff was recoverable from air sampled at heights up to 25 cm above the toilet seat. The highest numbers of C. diff were recovered from air sampled immediately following flushing, and then declined 8-fold after 60 min and a further 3-fold after 90 min. Surface contamination with C. diff occurred within 90 min after flushing, demonstrating that relatively large droplets are released which then contaminate the immediate environment. The mean numbers of droplets emitted upon flushing by the lidless toilets in clinical areas were 15-47, depending on design. C. diff aerosolisation and surrounding environmental contamination occur when a lidless toilet is flushed.
They concluded that lidless conventional toilets increase the risk of C. difficile environmental contamination, and they went onto suggest that their use should be discouraged, particularly in settings where C. diff is common.
In a 2018 study particle and bioaerosol concentrations were measured in hospital bathrooms across three sampling conditions; no waste no flush, no waste with flush, and fecal waste with flush. Particle and bioaerosol concentrations were measured with a particle counter bioaerosol sampler both before and after a toilet flushing event at distances of 0.15, 0.5, and 1 m from the toilet for 5, 10, 15 min. The results showed that particle concentrations measured before and after the flush were found to be significantly different (0.3–10 μm). Bioaerosol concentrations when flushing fecal waste were found to be significantly greater than background concentrations (p-value = 0.005). However, the bioaerosol concentrations were not different across time (p-value = 0.977) or distance (p-value = 0.911) from the toilet, suggesting that aerosols generated may remain for longer than 30 min post flush. Toilets produce aerosol particles when flushed, with the majority of the particles being 0.3 μm in diameter. The particles aerosolized include microorganisms remaining from previous use or from fecal wastes. Differences in bioaerosol concentrations across conditions also suggest that toilet flushing is a source of bioaerosols that may result in transmission of pathogenic microorganisms.[23]
Unfortunately despite these findings it is still common practice in UK healthcare that toilets within clinical areas have no lid and the aerosol created continues to create a risk of infection within a healthcare environment.
It has also been found that the use of hand dryers, especially the increasingly common jet air dryers might have the potential for increasing the risk of aerosols. In a recent study undertaken by E Best et al it concluded that multiple examples of significant differences in surface bacterial contamination, including by faecal and antibiotic-resistant bacteria, were observed, with higher levels when JAD (jet air dryers) were present versus PT (paper towel) in washrooms. [24]
In conclusion, it is now understood that bio-aerosols containing harmful pathogens can come from a number of apparatus within healthcare premises including showers, taps, washbasins, sinks, and toilets. Hand dryers are also a potential cause for concern. I believe that in order to reduce the instances of people becoming sick and sometimes dying due to healthcare-acquired infections caused by such bio-aerosols, manufacturers should continue to innovate and design better products that reduce aerosol risk. These new designs need to ensure that aerosol production and contamination is minimised. In addition, there should be very careful consideration of when and where to site sanitary apparatus and the risks associated with toilets within an immunocompromised patient area.
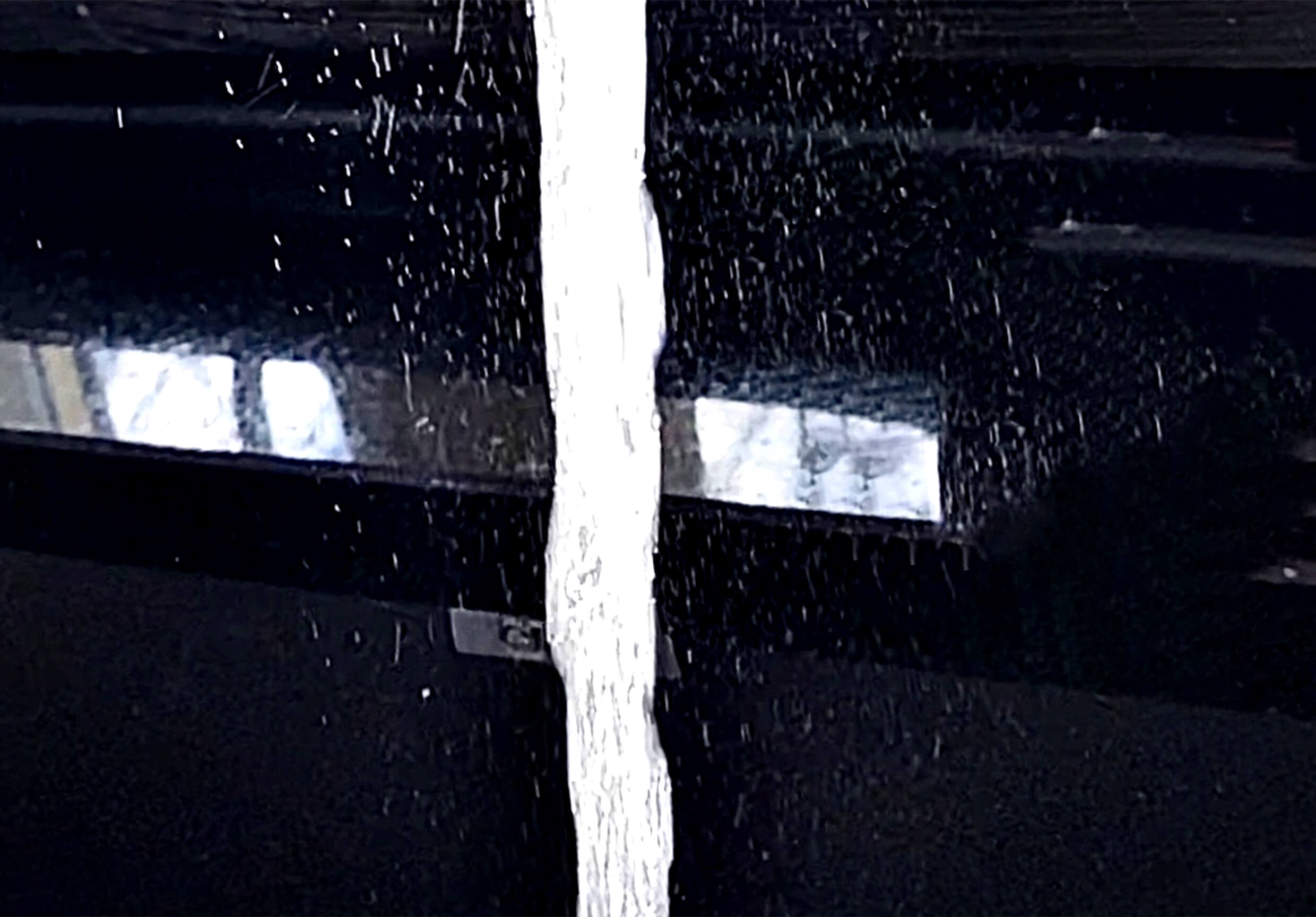
Water from a tap produces significant aerosol dispersal.

Toilet without a seat lid.

How Coronavirus is spread through aerosol through the air.
Image courtesy of Scientific American
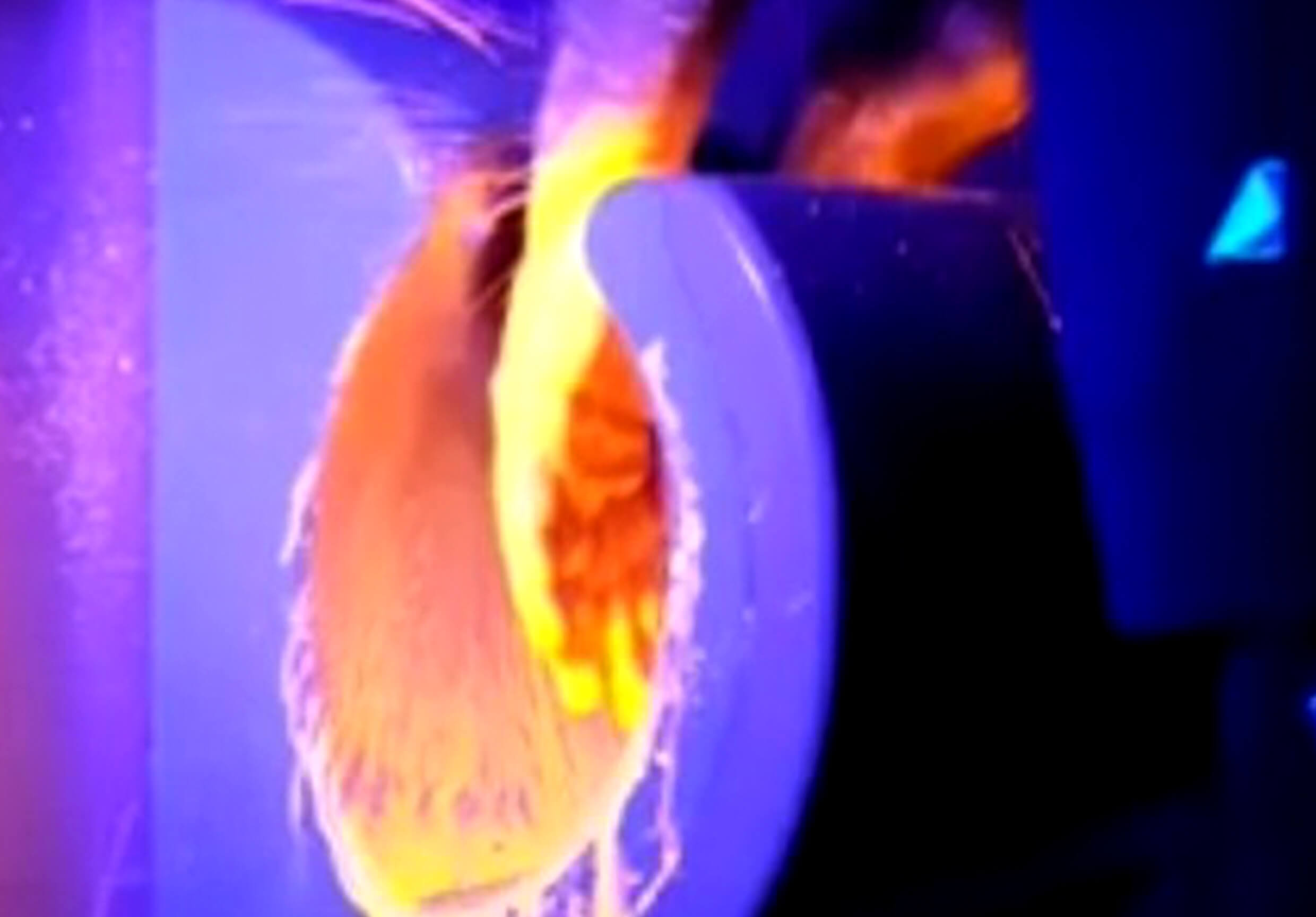
Picture of the aerosol dispersal caused by a hand dryer.
[1] Pittet D, Mourouga P, Perneger TV, and Members of the Infection Control Program. Compliance with hand washing in a teaching hospital. Ann Intern Med. 1999. 130:126–130.
[2] Tellier R., Li Y., Cowling B.J., Tang J.W. Recognition of aerosol transmission of infectious agents: a commentary. BMC Infect. Dis. 2019;19 doi: 10.1186/s12879-019-3707-y. [PMC free article] [PubMed] [CrossRef] [Google Scholar]
[3] Morawska L., Cao J. Airborne transmission of SARS-CoV-2: the world should face the reality. Environ. Int. 2020;105730 doi: 10.1016/j.envint.2020.105730. [PMC free article] [PubMed] [CrossRef] [Google Scholar]
[4] Judson S.D., Munster V.J. Nosocomial transmission of emerging viruses via aerosol-generating medical procedures. Viruses. 2019;11:940. doi: 10.3390/v11100940. [PMC free article] [PubMed] [CrossRef] [Google Scholar]
[5] Tang, J. W., Li, Y., Eames, I., Chan, P. K. S., & Ridgway, G. L. (2006). Factors involved in the aerosol transmission of infection and control of ventilation in healthcare premises. Journal of Hospital Infection, 64(2), 100–114.
[6] ASHRAE. ASHRAE position document on airbone infectious diseases. 2014.
[7] Barker J, Jones MV. The potential spread of infection caused by aerosol contamination of surfaces after flushing a domestic toilet. J Appl Microbiol. 2005;99(2):339–47. doi: [PubMed]
[8] WHO. Infection prevention and control measures for acute respiratory infections in healthcare settings: An update. 2007:39–47. [PubMed]
[9] Szymanska J. Dental bioaerosol as an occupational hazard in a dentist’s workplace. 2007:203–7. [PubMed]
[10] Laheij AM, Kistler JO, Belibasakis GN, Valimaa H, de Soet JJ, European Oral Microbiology W. Healthcare-associated viral and bacterial infections in dentistry. J Oral Microbiol. 2012;4. [PMC free article] [PubMed]
[11] Brankston G, Gitterman L, Hirji Z, Lemieux C, Gardam M. Transmission of influenza A in human beings. The Lancet Infectious Diseases. 2007;7(4):257–65. doi: 10.1016/S1473-3099(07)70029-4 [PubMed]
[12] Tellier R. Aerosol transmission of influenza A virus: a review of new studies. J R Soc Interface. 2009;6Suppl 6:S783–90. [PMC free article] [PubMed]
[13] Gralton J, Tovey E, McLaws ML, Rawlinson WD. The role of particle size in aerosolised pathogen transmission: a review. J Infect. 2011;62(1):1–13. doi: 10.1016/j.jinf.2010.11.010 [PubMed]
[14] Tuttlebee CM, O’Donnell MJ, Keane CT, Russell RJ, Sullivan DJ, Falkiner F, et al. Effective control of dental chair unit waterline biofilm and marked reduction of bacterial contamination of output water using two peroxide-based disinfectants. J Hosp Infect. 2002;52(3):192–205. [PubMed]
[15] Roberts K., Smith C. F., Snelling A. M., Kerr K. G., Nafield K. R., Sleigh P. A., Beggs C. B. 2008. Aerial dissemination of Clostridium difficile spores. BMC Infect. Dis. 8, 7. [PMC free article] [PubMed]
[16] Cordes, L. G., A. M. Wiesenthal, G. W. Gorman, J. P. Phair, H. M. Sommers, A. Brown, V. L. Yu, M. H. Magnussen, R. D. Meyer, S.S. Wolf, K. N. Shands, and D. W. Fraser. 1981. Isolation of Legionella pneumophila from hospital shower heads. Ann. Intern. Med. 94:195-197.
[17] P. L. Meenhorst A. L. Reingold G. W. Gorman J. C. FeeleyB. J. van Cronenburg C. L. M. Meyer R. van Furth. Legionella Pneumonia in Guinea Pigs Exposed to Aerosols of Concentrated Potable Water from a Hospital with Nosocomial Legionnaires’ Disease, The Journal of Infectious Diseases, Volume 147, Issue 1, 1 January 1983, Pages 129–132, https://doi.org/10.1093/infdis/147.1.129
[18] Garye Bollin, Joseph F.Plouffe, Michael F. Para and Barbara Hackman. Aerosols containing Legionella pneumophila generated by shower heads and hot-water faucets. Youngstown Hospital Association, Youngstown, Ohio 44501,1 and Department of Medicine, Division of Infectious Diseases, The Ohio State University Medical Center, Columbus, Ohio 432102 Received 2 May 1985/Accepted 10 August 1985
[19] Deborah De Geyter, Lieve Blommaert, Nicole Verbraeken, Mark Sevenois, Luc Huyghens, Helena Martini, Lieve Covens, Denis Piérard, and Ingrid Wybo
The sink as a potential source of transmission of carbapenemase-producing Enterobacteriaceae in the intensive care unit Antimicrob Resist Infect Control. 2017; 6: 24. Published online 2017 Feb 16. doi: 10.1186/s13756-017-0182-3
[20] Jessen CU. Airborne microorganisms: occurrance and control. Copenhagen: G.E.C. Gad Forlag; 1955.
[21] Barker J, Jones MV. The potential spread of infection caused by aerosol contamination of surfaces after flushing a domestic toilet. J Appl Microbiol. 2005;99:339–47. [PubMed]
[22] Best EL, Sandoe JA, Wilcox MH. Potential for aerosolization of Clostridium difficile after flushing toilets: the role of toilet lids in reducing environmental contamination risk. J Hosp Infect. 2012 Jan;80(1):1-5. doi: 10.1016/j.jhin.2011.08.010. Epub 2011 Dec 2.
[23] Knowlton, S.D., Boles, C.L., Perencevich, E.N. et al. Bioaerosol concentrations generated from toilet flushing in a hospital-based patient care setting. Antimicrobial Resistance Infection Control 7, 16 (2018). https://doi.org/10.1186/s13756-018-0301-9
[24] E. Best, P. Parnell, J. Couturier, F. Barbut, A. Le Bozec, L. Arnoldo, A. Madia, S. Brusaferro, M.H. Wilcox Environmental contamination by bacteria in hospital washrooms according to hand-drying method: a multi-centre study Journal of Hospital Infection, Volume 100 Issue 4, December 2018 (pages 469-475)
Author: Elaine Waggott (AMRSPH)

Tel: 07772 457497
email: [email protected]
Elaine began her working career working in the building and construction sector and co-owned a distribution company by the age of 24.
Elaine first worked within the plumbing industry In 2000 where she headed up a 60 person team responsible for customer service and technical support across the UK for Ideal Standard/Armitage Shanks leading several large projects within the company and using her experience from customer service, became involved in the process of new product development. After moving back to her home country of Scotland, she continued her career with Ideal Standard, running the business development team within Scotland for Ideal Standard/Armitage Shanks.
Over the last three years Elaine has been fully involved in the setting up of both Angel Guard and Water Kinetics with both of these companies having just launched cutting edge and highly innovative plumbing products. She currently holds the position of Director of Operations for both of these companies.
Elaine is Six Sigma trained, has written articles for newspapers and magazines, been editor for a leadership magazine, and has done both television and radio work. Elaine has extensive experience in public speaking and has recently been awarded membership of the Royal Society of Public Health.
